The Science Behind Cricket: A Crash Course by The CricketKeeper
- The Cricket Keeper
- Apr 7
- 13 min read
07.04.2025
When I was a kid, I used to say, “I want to be a doctor.” That ambition lasted until I was about 13, when I realised I absolutely hated science. I understood it well enough, but I could never bring myself to focus in class. It just didn’t interest me. I was far more interested in sports. So, I just counted down the days until I was free from science after my IGCSEs.
And yet, here I am, five years later, writing about the science behind cricket. The only way to get me to pay attention to science was to connect it to sports. I realised this because I chose PE as an IGCSE. Funny enough, the theory we learnt in PE was almost identical to what we covered in biology, but it actually made sense to me. And before anyone suggests it was because of the teachers—no, that wasn’t it. Both my PE and biology teachers were great. The difference was simple: in PE, everything was explained through sports, and that made it infinitely more interesting. So, children, come along with me as I take you on a ride to the world of science.
What do jetpacks and swing bowling have in common?
That might sound like a nonsensical question, but bear with me. In the same way that a jetpack would propel a person forward, a fast bowler uses a rapid run-up to carry speed through the final stride, transferring all that energy into their shoulder, arm, and then the ball. This is a simplified way of thinking about the science behind pace bowling, and that science is basically physics at work.
Physics sits at the heart of nearly everything that happens on a cricket field. The ball, the bat, the bounce off the pitch, the spin that a cunning bowler might impart, the trajectory of a lofted cover drive—behind all these is a blend of motion, force, and energy. Many of us find the equations that define these forces either intimidating or tedious, but when we see them play out in a real-life game, they’re suddenly intriguing. Momentum, for example, is simply mass multiplied by velocity. A cricket ball might only weigh around 156 grams, but if it’s hurtling towards you at 145 kilometers per hour, that small mass multiplied by its speed carries a real punch. The bowler has sprinted in from maybe 20 yards away, building pace, planting the front foot, and whipping the bowling arm over in a carefully coordinated motion. By the time the ball releases from the hand, the speed can be truly frightening. All that momentum is then taken by the batter’s bat or, sometimes less pleasantly, by the batter’s body. Understanding momentum through a jetpack analogy helps me grasp why something small can pack a huge punch if propelled fast enough.
The Jetpack Analogy: How Airflow Moves a Cricket Ball
Imagine you strap on a jetpack with two exhausts, each releasing flames with equal force. If both exhausts are firing evenly, you’ll shoot straight up. Now, let’s say you turn off the left-side exhaust while leaving the left one on full blast. You won’t hover or stay in place; you’ll swerve off toward the left. The thrust is unbalanced, so you tilt in the direction of the weaker side. A cricket ball that swings is basically doing the same thing in the air—except instead of rocket thrust, it’s airflow around the ball that’s unbalanced. One side of the ball experiences a more turbulent airflow, and the other side is smoother. This difference in airflow leads to a difference in pressure that forces the ball to bend or swing toward the side with lower pressure.
If you can control which side is smooth and which side is rough, plus the angle at which you hold the seam, you’re essentially deciding how your “jetpack” will tilt the flight of the ball. That’s why we see bowlers meticulously polishing one side of the ball and letting the other scuff up. They’re creating a controlled imbalance in airflow to make the ball swing.
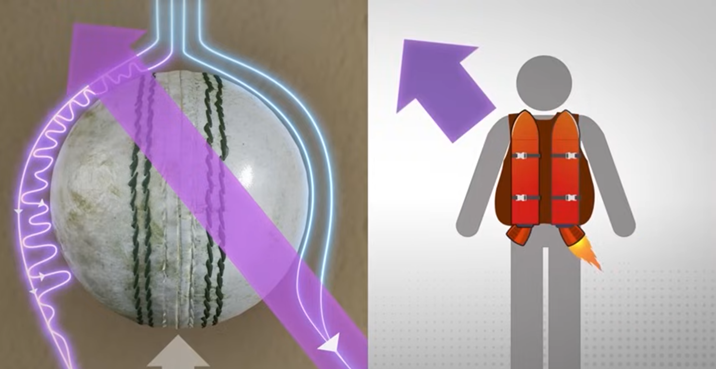
The Anatomy of a Cricket Ball: What Makes Swing Possible?
To truly appreciate swing, it helps to understand how a cricket ball is put together. A traditional Test match cricket ball has four pieces of leather, stitched around a core of cork and tightly wound string. The stitching forms a raised seam that is a crucial aerodynamic feature. When a fast bowler delivers the ball, the seam can disrupt airflow, and that disruption is what leads to lateral movement in the air (swing) or off the pitch (seam).
Over the course of a game, one side of the ball gets roughed up while the other side stays shiny if the fielding side polishes it. That difference in surface texture is what allows swing bowlers to manipulate airflow. As the ball gets older, you might see it reverse swing too, which can happen when the rough side becomes so uneven that the usual airflow patterns invert.
How Swing Actually Works: The Science of Airflow
The simplest explanation for swing is that air flowing over one side of the ball behaves differently from the other side. On the shiny side, the airflow is mostly laminar, which means it follows the contour of the ball smoothly. On the rough side, it goes turbulent, separating from the ball’s surface earlier. Because airflow stays attached longer on the smooth side, it moves faster and creates a slightly lower pressure than on the rough side. That pressure difference tugs the ball toward the rough side. In an outswinger to a right-handed batter, for instance, the shiny side faces the batter, the rough side faces the slips, and the ball curves away. For an inswinger, it’s reversed.
Much like our jetpack tilting, the cricket ball veers toward the side that’s less aerodynamically efficient. Bowlers who master releasing the ball at the perfect seam angle, with the right wrist position, can get the ball to swing late or early, depending on what they want. Late swing is especially lethal, because the batter has less time to readjust.
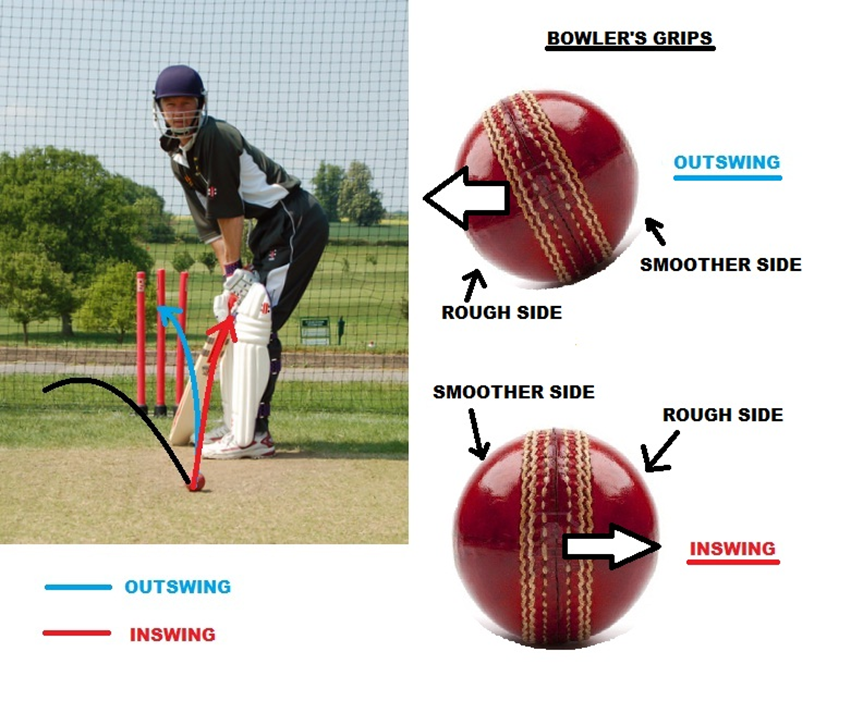
Spin Bowling: The Magnus Effect
Spin relies on something called the Magnus effect. A spinning ball drags air around it. The side that’s rotating in the same direction as the oncoming air will have one airflow pattern, while the side rotating against it will have another, creating a sideways force that bends the ball’s path in the air. A crafty leg-spinner, for instance, can make the ball drift slightly toward the leg side before it bounces and then spin sharply in the opposite direction. It looks magical, but there’s real science behind it. The bowler’s body action, wrist position, and finger snap are just as critical. Without a good pivot at the crease or strong shoulders to generate revolutions, you won’t get the flight and spin that trouble world-class batters.
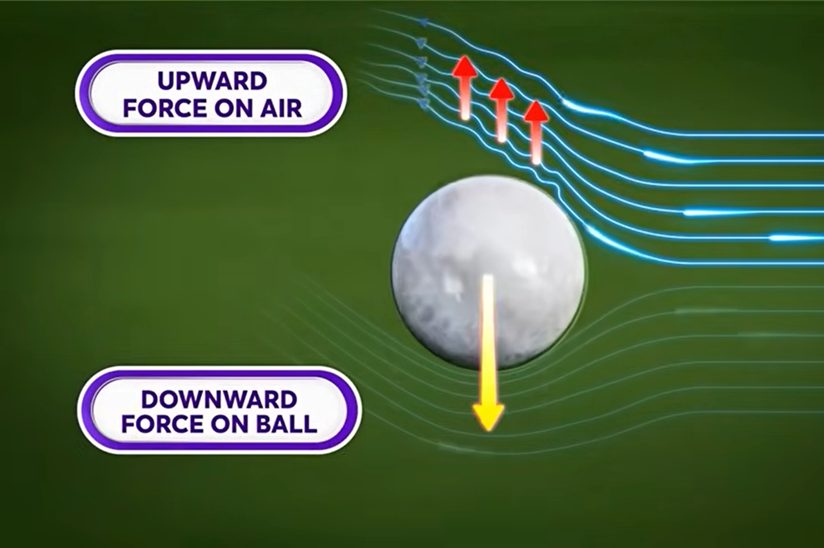
Biomechanics: The Body in Action
Pace bowlers rely on a kinetic chain that starts from the feet pushing off the ground and ends with the ball leaving the fingertips. If you watch it frame by frame, you’ll see the legs driving, the hips rotating, the torso twisting, the shoulder snapping around, and finally the wrist flicking. Each element builds on the previous one in a whip-like chain. If any link in that chain is weak or misaligned, you lose speed and risk injury. That’s why many pacers develop back problems; the bowling action is a high-stress motion repeated hundreds of times in a match or in training. Strengthening the core, hips, and shoulders can help hold the body in the right positions to safely channel that energy.


Spin bowling might look less physically intense, but it has its own demands. Good spinners often have a powerful pivot on the front foot and a quick torso rotation. The arm and wrist generate the majority of the spin, but the rest of the body sets the stage for that flourish at the end. They also need a cunning mind to outfox batters, disguising variations like googlies or carrom balls. You can know all the science in the world, but if you telegraph your variation, a sharp-eyed batter will pick it right away. So even here, science meets trickery, a dance of physics and psychology.
Batters have their own biomechanics to master. A solid batting technique involves a stable base, moving your feet to the ball, and swinging the bat with fluid coordination of arms, shoulders, and torso. Coaches talk about getting your head over the ball, which might sound vague until you realise it helps maintain balance and ensures your eye level doesn’t waver mid-shot. When everything lines up perfectly, the energy from your body travels into the bat, and then into the ball, sending it racing away. Hit the ball off-center, however, and you’ll lose power or slice it unexpectedly. That’s why edges happen, often leading to a batter’s downfall. The science here is straightforward: the bat’s sweet spot is designed to give the best transfer of energy, so if you connect there, the ball travels quickly. Strike too close to the edge, and some of that energy goes into twisting the bat or creating vibrations, rather than launching the ball where you want it to go.
The Pitch and Weather: Nature’s Influence
The pitch itself is another dimension of science in cricket. Groundskeepers work meticulously on soil composition, grass coverage, rolling, and watering to produce a specific type of playing surface. A hard, dry pitch often gives more bounce and pace, while a greener pitch can aid seam movement. A crumbly, dusty surface tends to favor spin, allowing the ball to grip and turn. Over the course of a multi-day match, the pitch might deteriorate, developing cracks or patches that behave unpredictably. This ongoing evolution forces players to constantly adjust. It’s one reason Test cricket can be so engrossing: the conditions aren’t static. Day one might be great for batting, but by day four or five, spinners come into their own. The same piece of turf has undergone a transformation, and that shift is rooted in a blend of biology (grass growth), physics (compression underfoot, dryness in heat), and chemistry (moisture evaporation).
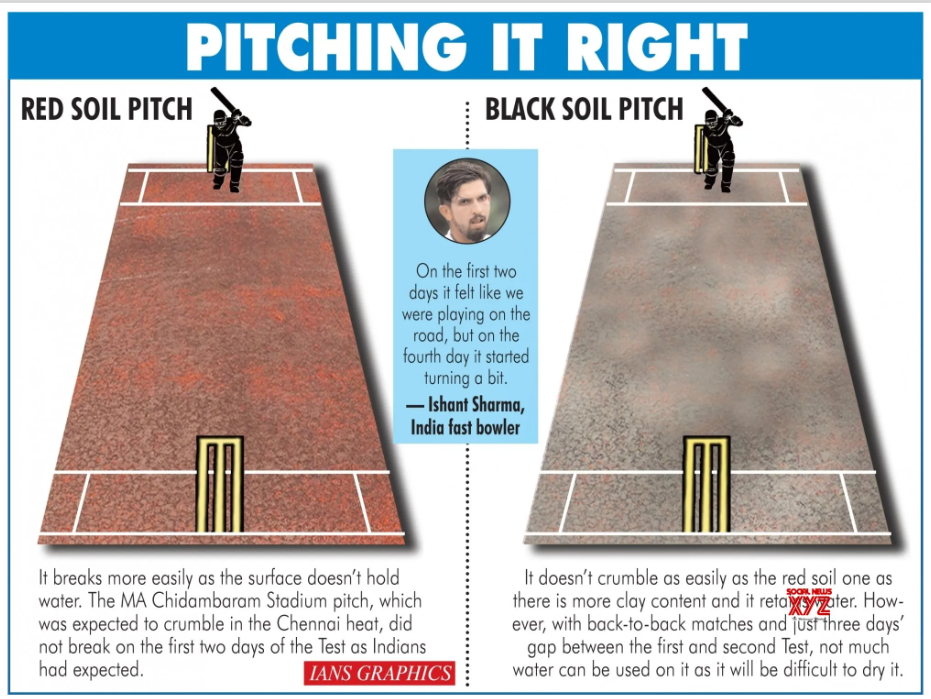
Then there’s the weather. Cricket is famously a sport that pauses for rain, but less obvious aspects of weather also matter. Cloud cover, temperature, and humidity can influence how the ball swings. Some say a cloudy sky helps the ball move more, though scientific studies suggest it might be related to air density, temperature, or the micro-changes in the ball’s surface when there’s moisture in the air. Wind, too, can give a bowler an advantage, pushing the ball in one direction or letting them run in faster. High-altitude venues, where air is thinner, can reduce swing but help the ball travel farther off the bat. Each of these environmental factors layers on another piece of the cricket puzzle, so the game becomes a lively interplay of meteorology, aerodynamics, and pure skill.
Another intriguing area is how different parts of the world produce different styles of cricket, partly due to local pitches and climates. In Australia, bouncy pitches favor back-foot play and tall fast bowlers who hit the deck hard. In England, the damper conditions and grassy pitches encourage swing bowlers and batters who play the ball late with soft hands. In the subcontinent, lower, dustier surfaces offer turn, so batters there grow up mastering spin. These regional preferences have shaped legends of the game: think of Australian fast-bowling greats, English swing masters, and Indian spin kings. Even within a single country, the micro-climate of a stadium or the composition of its soil can give it a reputation for favoring certain types of bowlers. It’s like each pitch is a mini-ecosystem with its own rules, and cricketers are the
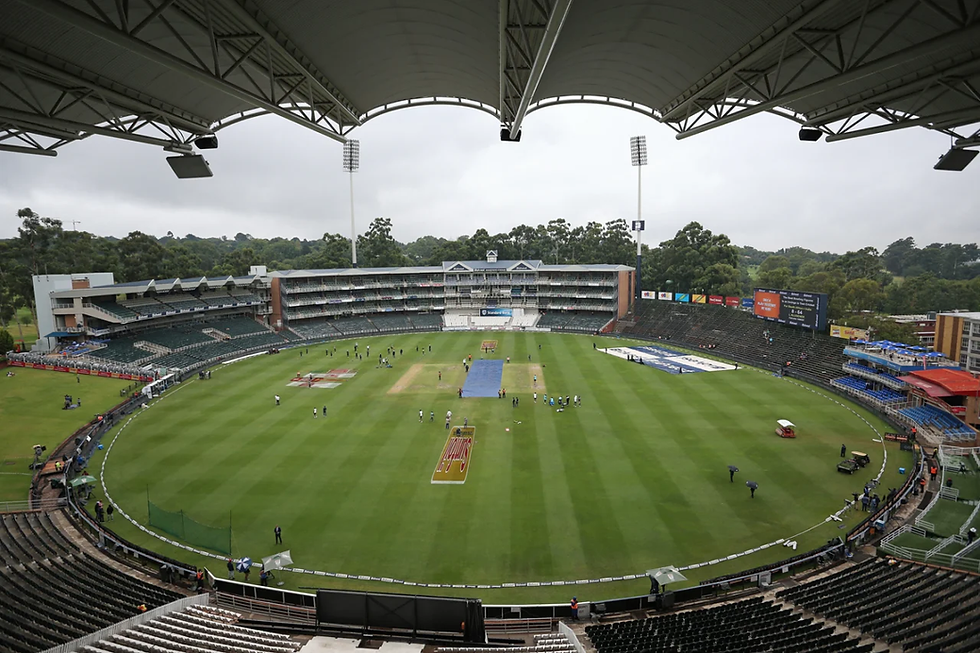
At altitude, such as in Johannesburg, the thinner air means the ball travels faster, and batters might hit more sixes because there’s less drag. That same thin air can reduce swing. Conversely, bowlers might bowl a fraction quicker, but lose the sideways movement that a normal-density atmosphere would grant. If you compare a match at sea level in a humid coastal region versus one played in a high-altitude stadium, you’re basically comparing two distinct laboratories. The game doesn’t change, but the conditions add or subtract variables.
The Mental Factor: Reaction and Strategy
Mental science is also huge in cricket. A fast bowler can release the ball in around half a second from jump to landing. The batter has to pick up cues about speed, line, length, even seam orientation in that fraction of a second. Elite players develop almost preternatural reflexes and anticipation, using a combination of muscle memory and pattern recognition to make decisions rapidly. A slip fielder might have even less time to react to a sharp edge. They rely on reflex catching, which involves letting the ball’s momentum slow by moving the hands back—a tiny biomechanical trick that reduces the chance of the ball popping out. Beyond reaction, there’s also strategy and psychology. A batter might try to dominate a spin bowler early to break their confidence. A bowler might pepper a batter with short balls to push them onto the back foot. Captains set clever fields, reading not only the batter’s strengths but the pitch conditions, weather, and how the ball might behave. It’s a chess match played at high speed, and it’s all about decision-making under pressure.
Modern Technology: Data Meets Drama
If you think that’s enough science to fill a textbook, you’d be right. But there’s more. Technology has upped the ante. From motion-capture cameras analysing bowling actions to ball-tracking systems predicting trajectories, modern cricket is awash in data. The Decision Review System uses principles from physics and computer vision to show whether a ball pitched in line or would have hit the stumps. Hot Spot relies on thermal imaging to detect contact. Snickometer or UltraEdge uses audio frequencies to pick up the tiniest nicks. All these systems add layers of objectivity, and yet the game still retains its unpredictability. Even the best technology can’t account for a sudden gust of wind or a freak bounce off a crack in the pitch.
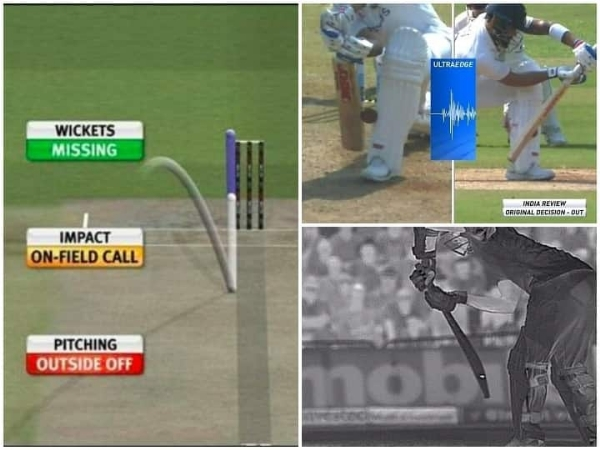
Strength and Conditioning
Strength and conditioning also tie heavily into sports science. Professional players train not just to build muscles but to fine-tune the exact type of strength or flexibility they need. Fast bowlers often do exercises that mimic their action, focusing on explosive power in the legs, rotational strength in the core, and shoulder stability. Spin bowlers work on wrist and finger strength, shoulder mobility, and balance. Batters spend time in nets trying to groove their muscle memory, so that judging line and length becomes instinctive. Fielders run catching drills that train their reflexes and hand-eye coordination. All of this is guided by knowledge about how muscles adapt, how the brain learns new motor patterns, and how to recover properly to prevent injuries.
Cricket is also unique in how long matches can last. A five-day Test can be a war of attrition, not just a burst of speed. Bowlers have to conserve energy, captains need to time their bowling changes, and batters must remain mentally focused for hours on end. That kind of endurance ties into physiology. Your body needs a steady supply of glucose and oxygen to keep performing at a high level. Fatigue can set in, affecting concentration and coordination. Even the best technique can degrade if you’re too tired to maintain it. That’s why sports scientists and nutritionists play a growing role, ensuring players stay hydrated, fuelled, and sufficiently rested. It’s easy to overlook, but in scorching conditions, players can lose a lot of fluids, which impacts both mental clarity and muscle function.
The Pink Ball in Day-Night Tests
The day-night Test format introduced the pink ball, which is supposed to be more visible under floodlights. But the pink ball also behaves slightly differently, both in how it swings and how it scuffs. Some players claim it swings more under lights, others say it’s harder to pick up as a batter. These are areas where anecdotal evidence meets the quest for measurable data. Manufacturers experiment with dyes, coatings, and stitching techniques to find the ideal balance. It’s a reminder that even one change in the ball’s materials can ripple through the entire match experience.
T20 Cricket: A Lab for Rapid Innovation
Meanwhile, T20 cricket compresses the whole scientific drama into a short, frantic spectacle. Momentum swings can happen over a couple of overs, and batters often take on outrageous shots that test the limits of biomechanics. Scoops, ramps, and switch-hits are not just crowd-pleasing stunts; they’re demonstrations of a player’s adaptability. A bowler might experiment with slower bouncers or knuckle balls, each requiring a different grip and wrist action to manipulate air resistance. If you stretch the imagination, you could say T20 is a lab for immediate results: whatever you try, you see the outcome within seconds rather than over days. It’s a perfect environment for rapid innovation, which is partly why T20 led to new inventions in the game.
Protective Gear
Protective gear highlights another side of science in cricket. Modern helmets have advanced shells and padding designed through rigorous testing. The foam or padding inside can dissipate impact forces, reducing the risk of serious injuries when a ball smashes into the grill at speed. Batting pads, arm guards, and thigh pads are similarly engineered, using layered materials that spread out the kinetic energy of a fast-moving ball. In older eras, players went without helmets, which, looking back, was a massive risk, especially against the lightning pace of some West Indian quicks from the 1970s and 80s. Over time, as we understood the dangers more deeply, technology evolved to protect players better. The quest for safety in sport is inextricably tied to scientific research, whether it’s about understanding force absorption or designing more ergonomic gear.
Embracing Complexity and The Human Factor
If you imagine a giant spreadsheet of all these factors; pitch hardness, soil composition, ball condition, air temperature, humidity, a player’s muscle fatigue, and so on—you realise just how complex cricket can be. Perhaps that’s why it captivates so many people for hours or days on end. There’s always a new variable to consider, a new trick to try, a new adaptation to make. Some people might find that complexity daunting or even boring, but for those who love the sport, it’s part of the narrative. Each session can offer a fresh twist because the pitch might have dried out a bit more, or a bowler might have figured out a new variation, or a batter has finally adjusted to the bounce.
Of course, the human factor can override all the science in a moment of brilliance or panic. A fielder might misjudge a catch despite perfect conditions, or a batter might middle a shot straight to a fielder. These moments remind us that cricket is played by people, not robots. Science can explain the setup, but it can’t guarantee the outcome. That’s the X-factor. A bowler’s form, a batter’s confidence, or a crowd’s energy can shift momentum in ways that data can’t fully predict. The psychological side is just as real, whether it’s a sudden loss of concentration leading to a reckless shot or a burst of adrenaline helping a bowler bowl the perfect yorker at the death.
There’s a lovely irony that someone like me, who dreaded science classes, can now be lyrical about laminar flow and biomechanics just because they happen to apply to cricket. Maybe it’s a lesson in how relevance can change our attitudes. Had someone told me back then that I’d be analysing how the seam position affects swing, I would have laughed and said, “I’d rather watch paint dry.” But once again, here I am. Thus, we end our marathon exploration of science in cricket.
Comments